Earlier this year I gave a presentation The Impact of AI and Robotics on Space Settlement, which was a successor to a presentation I first gave in 2019 at the International Space Development Conference. Titled Robotic Space Settlement, that presentation focused on the high cost differential between human-based versus robotic-based space operations and made the case that using robots rather than humans to create economic value for Earth from space operations would produce larger benefits at a lower cost in a shorter time frame.
I subsequently proposed that NSS host a Space Forum in the form of a debate between NSS Space Ambassadors on this subject. That debate was held on 11/30/2023. Titled Space Development is Best Left to AI-Enhanced Robots, the debate featured myself arguing for robotic development, Stevan Akerley arguing for human development, and Bryce Meyer providing commentary on the state of robotics and AI (Artificial Intelligence).
The purpose of this essay is to provide background information on aspects of the arguments I incorporated into my two presentations and the space forum debate. The emphasis is on the proposition that the construction and deployment of a Space Solar Power satellite system should not be dependent on the availability of a human work force that has as its home a L5 space colony. This essay is organized into the following sections:
- Introduction: Malthus Versus O’Neill
- The Case for Space Settlement
- Freeman Dyson’s Space Colony Analysis
- International Space Station Versus Island One Space Colony
- The Challenges of Building A Space Colony
- The Fourth Industrial Revolution and Robots
- Using Robots or Astronauts to Explore Mars
- Human vs Robotic Economics
- Conclusion
Introduction: Malthus Versus O’Neill
Our story begins some 50 years ago at a time when Malthusian predictions were painting a grim picture for humanity’s future. The book The Population Bomb was predicting a future of mass starvation while calling for a number of grim mandatory controls to be imposed by governments on people’s ability to reproduce. More influential, and rational, was the 1972 book The Limits to Growth which used a world model to predict that the world’s supply of petroleum would be gone by 2000. That model also projected that our gold supply would be exhausted by the early 1980’s. Supplies of copper, lead, natural gas, silver, tin, and zinc were also all predicted to have been exhausted by now.
The world model used in The Limits to Growth was based on five variables: population; food production; industrialization; pollution; and consumption of nonrenewable natural resources. According to the book’s model:
- Global industrial output per capita would peak around 2008, followed by a rapid decline
- Global services per capita would peak around 2020, followed by a rapid decline
- Global food per capita would peak around 2020, followed by a rapid decline
- Global population would peak in 2030, followed by a rapid decline
At about the same time, new solutions to Earth’s problems were being proposed. These solutions called for using outer space to open up our world system for access to both new physical space and new sources of raw materials, especially metals. The dean of this vision was Princeton University physicist Gerard O’Neill. His book The High Frontier: Human Colonies in Space was highly influential and opened our minds to a new way of seriously thinking about our place in the cosmos.
O’Neill’s book was preceded by his 1974 article The Colonization of Space which appeared in Physics Today, In that article, O’Neill provided a detailed review of the parameters and cost of construction for a space colony at L5 (L5 being one of the five Lagrange points in the Earth-Moon system) and concluded that an initial space colony could be built within 20 years. The article also predicted that by around 2050 emigration to space colonies could reverse the rise in Earth’s population and that by 2100 the total land area in the colonies would be more than three times that of Earth. O’Neill viewed this as resulting in nearly all of Earth’s industrial activities being moved away from the Earth’s fragile biosphere, thus sparing our biosphere from the various stresses mining and industrial activities impose on it.
Conclusion: Space development can be used to provide humanity with access to a new array of economic resources.
The Case for Space Settlement
There have been many reasons given for pursuing space settlement or space colonization. These reasons include:
- access to new sources of energy (Space Solar Power, Lunar Helium-3)
- access to new sources of raw materials (natural resources), especially metals
- access to new physical space
- to ensure humanity’s, and life’s, long term survival by spreading life throughout the solar system
- to save the Earth’s natural environment by moving manufacturing off-planet
- to better protect the Earth from the asteroid and comet impact threat (commonly referred to as planetary defense)
- to make use of the zero-g environment of space for both research and the manufacture of materials impossible to produce in Earth’s gravity environment
- to better study and understand the geology and history of our solar system
- to improve our ability to study the universe
- to develop new technologies that would benefit Earth
For purposes of this article, the focus is on the economic benefits that would accrue to the inhabitants of Earth with a focus on energy, zero-g manufacturing (more broadly referred to as ISAM or In-space Servicing, Assembly, and Manufacturing), access to extraterrestrial resources, and planetary defense.
Conclusion: There are many arguments supporting a program of space settlement. The challenge is to construct a convincing framework that demonstrates that the commitment of resources for such projects can be justified against alternative uses for those same resources.
Freeman Dyson’s Space Colony Analysis
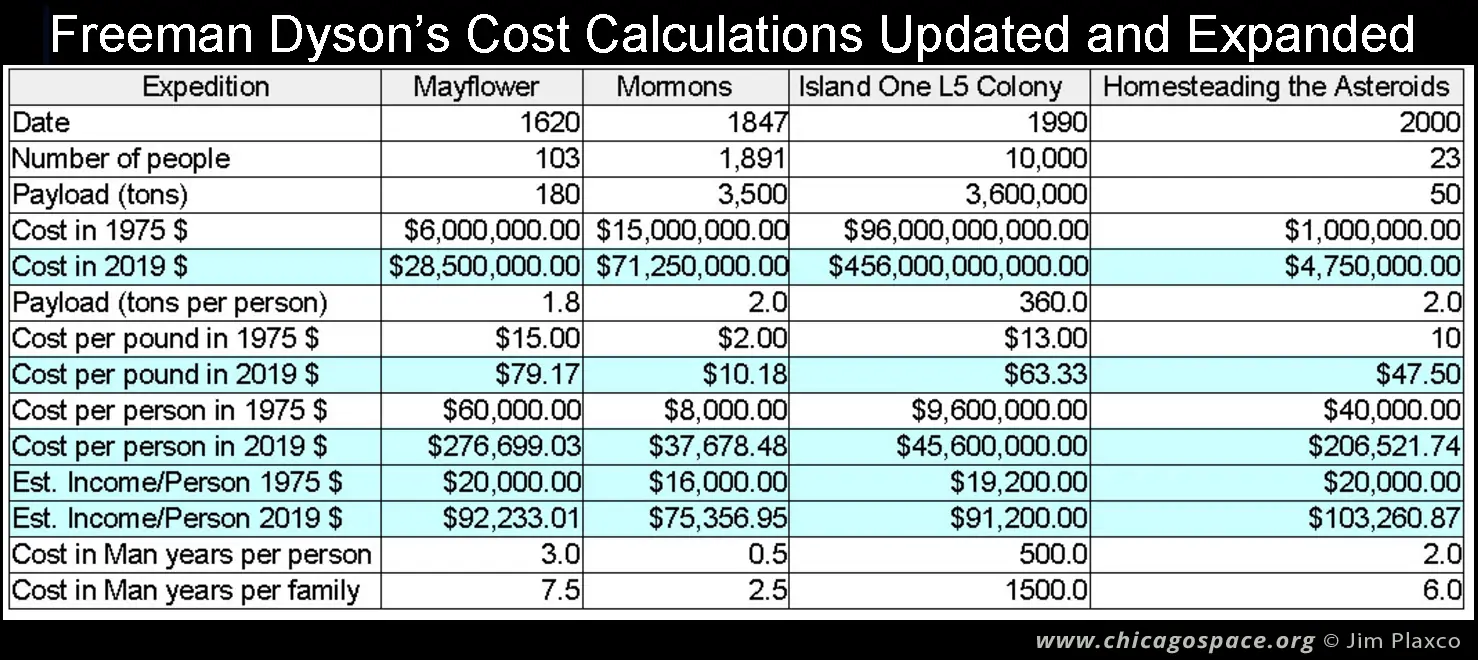
In 1978, the renowned theoretical physicist and mathematician Freeman Dyson addressed the question of space colonies in his essay Pilgrim Fathers, Mormon Pioneers, and Space Colonists: An Economic Comparison. The key question that Dyson asked was whether or not the inhabitants of a space colony could pay for the colony themselves, much as the Pilgrims and Mormons had done in previous centuries.
In the table shown in Figure 1, the rows in blue are a combination of my own updates to reflect 2019 dollar values versus the original 1972 dollar values and the addition of two rows that identify the estimated income per person for both 1975 and 2019 required to cover the project’s costs with respect to the cost in man-years of labor per person (total cost per person divided by the number of man-years of labor per person).
This is where the math paints a grim picture. Whereas a person on the Mayflower expedition could pay off their portion of the project’s debt with just 3 years of labor and a person on the Mormon expedition could cover their portion of the debt with 6 months of labor, it would take an inhabitant of O’Neill’s Island One Space Colony 500 years to pay off their share of the debt. Not considered in Dyson’s calculations was whether or not the economic value created each year by the colony’s workforce would be able to also cover the ongoing maintenance and operational costs of the space colony.
O’Neill saw the principle economic output of the colony’s population as being the construction of additional space colonies and, later, the construction of solar power satellites.
Conclusion: Given present levels of infrastructure, technology, experience, and the estimates of the expected cost per person for the construction of a space colony, an Island One Space Colony can not be self-financed solely by its inhabitants.
International Space Station Versus Island One Space Colony
To date, the best comparison we have for the design, engineering, orbital construction, and maintenance challenges a L5 space colony would present is the International Space Station (ISS).
For comparison, while ISS has a mass of approximately 460 tons, the Island One Space Colony would be some 7,800 times more massive at 3,600,000 tons. The Island One Space Colony would also be vastly more complicated than ISS – supporting a population of 10,000 men, women, and children rather than ISS’ rotating population of 6.
It was 26 years from the space station’s program announcement to construction complete – with some planned components never being built. Contrast this with O’Neill’s estimate that the Island One Space Colony could be built in 20 years.
Then there is the question of cost. The estimated cost of building and assembling ISS is on the order of $150 billion. Dyson’s estimated cost of construction for the Island One Space Colony (adjusted to 2019 dollars) is $456 billion – just three times more than the cost of ISS. While it can very reasonably and convincingly be argued that a commercially built and operated space station would have cost a fraction of the price of ISS, the mass, volume, complexity, and location of the Island One Space Colony would cost much more than either O’Neill’s or Dyson’s estimates.
Reinforcing the idea that the Island One Space Colony cost estimates were too low is a 2014 report from the National Academy of Sciences that estimated that the cost of a long surface stay conjunction class mission to Mars for four astronauts would cost between $300 and $600 billion.(2)
Conclusion: O’Neill’s and Dyson’s estimates of the cost to build an Island One Space Colony greatly underestimated those costs. It is also reasonable to conclude that the construction time estimates were also underestimated. These combine to greatly increase the difficulty of identifying products that can be cost competitive in Earth’s global market, thus hindering the ability of a space colony to be economically self-sufficient.
The Challenges of Building A Space Colony
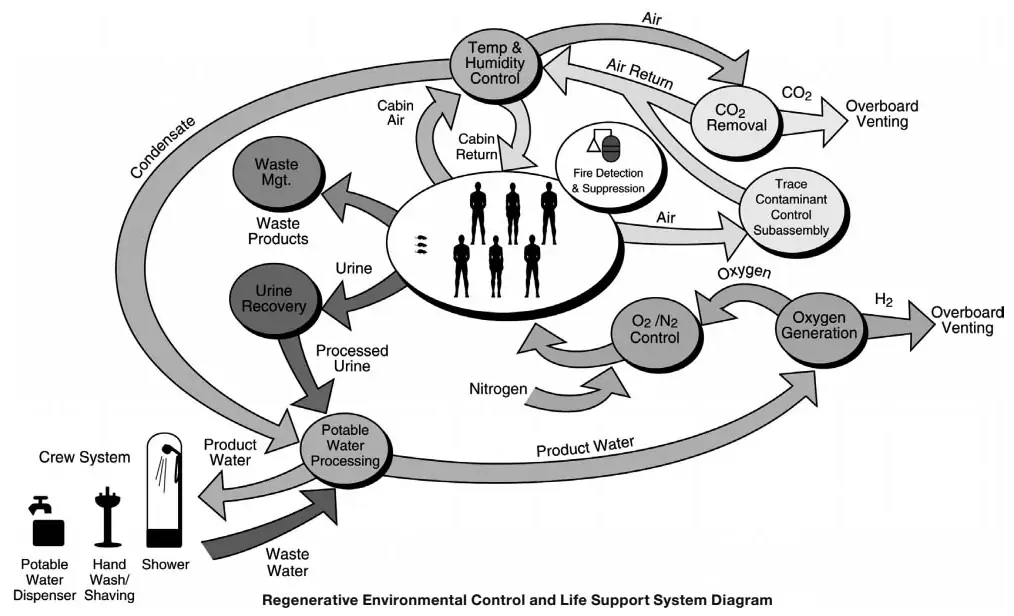
A principal technological challenge of space settlement is the creation and maintenance of an artificial environment that is suitable for life, be it human, plant, or animal. Exacerbating this challenge will be including the ability for the space colony to be self-sufficient when it comes to satisfying the basic needs of life.
System components of a space colony’s life support network would include:
- Air Supply, Purification, Distribution, Recycling
- Water Supply, Distribution, Recycling
- Thermal Management and Climate Control
- Food Supply, Management, Storage
- Energy Supply, Storage, Distribution,
- Waste Collection, Processing, Disposal
- Fire Detection/Control/Suppression Systems
- Radiation Shielding
- Debris Impact Shielding
- Shelters and Lifeboats
- Medical Infrastructure
This complex network of interconnected systems represents a significant engineering challenge. Contributors to the high cost nature of a space colony’s environment can be summarized as being:
- Immature TRL (Technology Readiness Level) that equate to increased levels of technological risk
- Complex interconnected systems in an extreme environment requiring extreme engineering
- Maximal levels of system reliability requiring extreme fault tolerant systems
- Initial and extended lack of economies of scale and scope
- Transportation costs associated with the import/export of materials and consumables
Given that such a system has never before been created, the design and construction of a large space colony would be the most complex undertaking in human history.
Conclusion: The construction of a self-sustaining space colony for 10,000 inhabitants is a massive undertaking that will require significant time to complete and will consume a significant amount of resources. Using as an example the deployment of a Solar Power Satellite system: the construction of a SPS system that is dependent on a space colony based human labor force will take much longer to build and incur significantly higher construction costs since the SPS construction is dependent upon the successful completion of the space colony construction. Chaining the costs of the space colony into the costs of the SPS system will significantly increase the price per kilowatt hour of electricity that must be charged to cover all system costs, thus making SPS generated electricity significantly less cost competitive. Alternatively, relying on automated assembly will reduce the time required to bring the system online as well as reducing the system’s assembly costs which combine to increase the probability that a SPS system can be cost competitive with Earth-based energy generation alternatives.
The Fourth Industrial Revolution and Robots
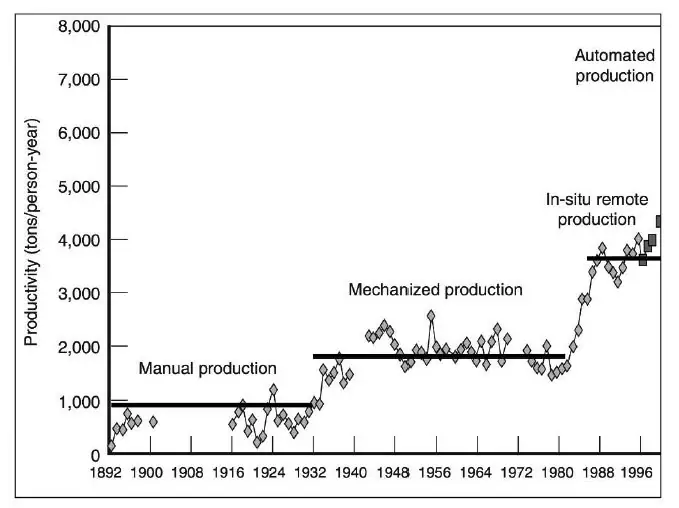
Society has undergone three industrial revolutions and is now said to be undergoing a fourth industrial revolution. The First Industrial Revolution occurred during the years 1760-1840 and saw the application of water power and steam power to drive mechanized production. The Second Industrial Revolution was from approximately 1870 to 1914 and involved the use of electric power to drive mechanized mass production. The Third Industrial Revolution dates from approximately 1947 to 2015 and involves the use of digital electronics and information technology to further automate production. As an illustration of mechanical innovation driving past productivity growth, Figure 3 above shows the successive steps in productivity growth for the mining industry over the course of the 20th century.
We are currently said to be in a Fourth Industrial Revolution whose birth dates to the end of the Third Industrial Revolution. The Fourth Industrial Revolution is a term coined by Klaus Schwab of the World Economic Forum in 2016 and involves the interconnectedness of digital devices (IoT or the Internet of Things) via networks and the use of cyber-physical systems to create smart factories.
A key product of this Fourth Industrial Revolution is the evolution of Lights-Out manufacturing where factories run in a fully autonomous mode without human intervention. Lights out manufacturing exclusively refers to production where machines are entirely self-sufficient. Also known as a “dark factory”, it’s a production method that requires little to no human interaction—essentially running on its own.
In his 2016 book The Fourth Industrial Revolution, Klaus Schwab wrote:
By enabling “smart factories”, the fourth industrial revolution creates a world in which virtual and physical systems of manufacturing globally cooperate with each other in a flexible way. This enables the absolute customization of products and the creation of new operating models.
The fourth industrial revolution, however, is not only about smart and connected machines and systems. Its scope is much wider. Occurring simultaneously are waves of further breakthroughs in areas ranging from gene sequencing to nanotechnology, from renewables to quantum computing. It is the fusion of these technologies and their interaction across the physical, digital and biological domains that make the fourth industrial revolution fundamentally different from previous revolutions.
Still valid today is the lesson from the first industrial revolution – that the extent to which society embraces technological innovation is a major determinant of progress.(3)
The advance of automation has been crucial to the ongoing improvement in our standard of living – with the principal challenge being the replacement of human labor with mechanized labor. In considering the role of automation in improving levels of productivity, a 2018 U.S. Census Bureau report Automation, Labor Share, and Productivity: Plant-Level Evidence from U.S. Manufacturing found that:
“The diffusion of automation is believed to be one of the fundamental drivers of both the decline in employment, and the increase in output and productivity in U.S. manufacturing over the past decades, during which labor’s share of output has also diminished.”(4)
Conclusion: Productivity growth boils down to being able to use less input to produce a given amount of output from one year to the next, It is the continued improvement in automation’s capabilities from innovations in computing power, algorithms, sensor technology, and the ability of machines to share information via networks (the Internet of Things) that is behind what has been termed the Fourth Industrial Revolution and the associated improvements in the capacity of automated systems to produce goods and services at ever lower cost.
Using Robots or Astronauts to Explore Mars
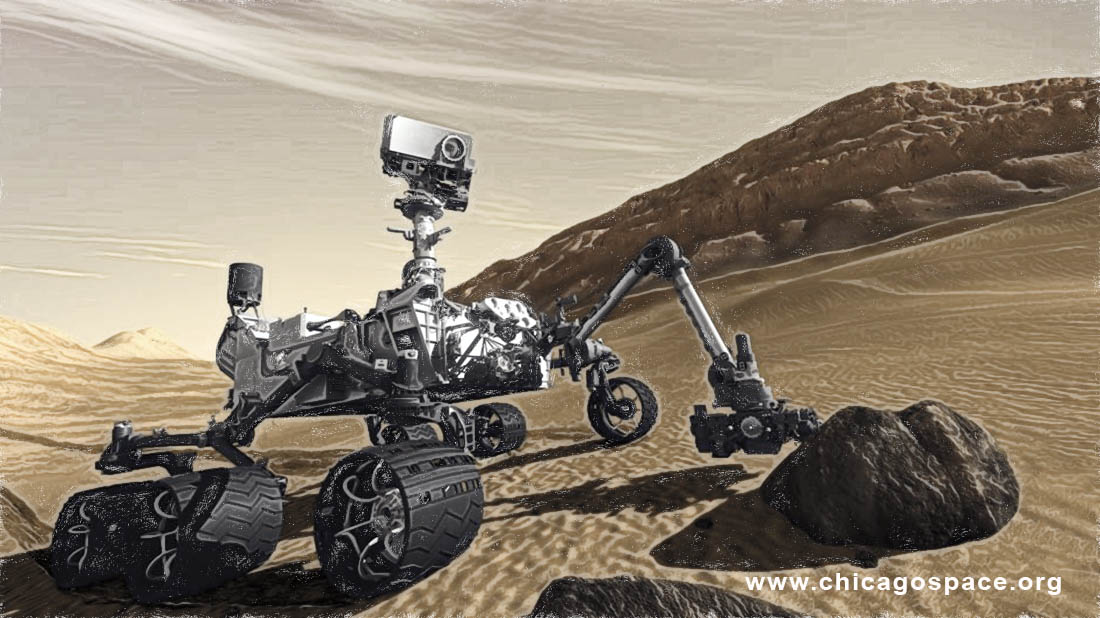
An example of the cost difference between human and robotic missions is to consider the cost challenges of human missions to Mars as compared to robotic missions, Dr. Steve Chien, a JPL Fellow, Senior Research Scientist, and Technical Group Supervisor of the Artificial Intelligence Group at NASA JPL (Jet Propulsion Laboratory) observed that “Sending humans even to low Earth orbit requires an amazing endeavour; sending humans to the Moon required an enormous endeavour; sending humans to Mars is even more challenging.”
Recall the 2014 National Academy of Sciences report (previously referenced) that estimated that the cost of a long surface stay conjunction class human mission to Mars would cost between $300 and $600 billion. While there have been no human missions to Mars because of the cost, there have been some 50 robotic missions to Mars, including missions by Japan, India, United Arab Emirates, and China.
On the subject of astronauts or robots for Mars exploration, in a 2019 interview former astronaut Steve Swanson compared the achievements of the Apollo astronauts on the Moon with those of the NASA Mars Curiosity rover. Swanson pointed out that the two Apollo 17 astronauts drove 11.6 kilometers/day while the Curiosity rover traveled an average of just 9 meters per day. Also that while Apollo 17 collected 247 regolith samples/day, Curiosity averaged only 0.013 regolith samples per day. This was at a cost in 2015 dollars of $20 billion for the Apollo 17 mission and $2.5 billion for the Curiosity mission. Elaborating on these points, Swanson stated that:
Yes, I am comparing a lunar mission to a Mars mission, which isn’t exactly fair. So, let’s use an estimate of what a human mission to Mars is expected to cost – the number ranges from around $100 billion to $500 billion per mission. I imagine it is going to be closer to the $500 billion or more given that the ISS alone cost more around $150 billion.
If we assume $500 billion per mission – a figure that would hopefully decrease with multiple missions – then a manned mission would yield a better return on the investment. From the ballpark estimates above, we can say a crew of four would be at least 500 times more productive in performing science than a rover, although the cost would be about 200 times greater.
If we assume $500 billion per mission (4 astronauts to Mars) we can say a crew of four would be at least 500 times more productive than a rover, although the cost would be about 200 times greater.(5)
It must be pointed out that a human mission to Mars will be on the surface for a very limited amount of time due to the limitations on the amount of resources (food, water, air, etc.) that they can bring with them and the need to make the return journey to Earth. An opposition class human mission to Mars would limit the astronauts to just 30 days on Mars. Alternatively, with a conjunction class human mission to Mars the astronauts would be able to spend 550 days on the planet. Whether it is an opposition class mission or a conjunction class mission, I have no doubt that astronauts would be more productive on a per day basis. But robots are not limited in that regard. The NASA Curiosity mission was launched on its trip to Mars on Nov. 26, 2011 and landed on the red planet on Aug. 6, 2012. Curiosity has now been active for over 4,000 days on Mars and has taken over 1.1 million photographs in that time and is still going strong having now traveled more than 30 kilometers across the Martian surface – surpassing the distance traveled by the Apollo 17 astronauts.
There is also the question of the availability of capital resources to fund missions to Mars. While $2 billion for a robotic mission to Mars amounts to a mere 6 percent of NASA’s 2023 budget, the cost of a human mission to Mars using Swanson’s estimate of $500 billion would be more than 16 times (1,612%) larger than NASA’s total 2023 budget.
Lastly, Swanson’s comparison ignores robotic evolution. Each succeeding generation of rover that we have sent to Mars has been more capable than the rovers that went before it.
Conclusion: The scale of capital requirements to support human missions in comparison to robotic missions must be addressed for any economic-centric space projects, whether its space-based solar power, zero-g manufacturing, or mineral extraction. It should be understood that the larger the amount of capital required to implement a project and the greater the amount of time to achieve project completion, the less likely that project is to be funded.
Human vs Robotic Economics
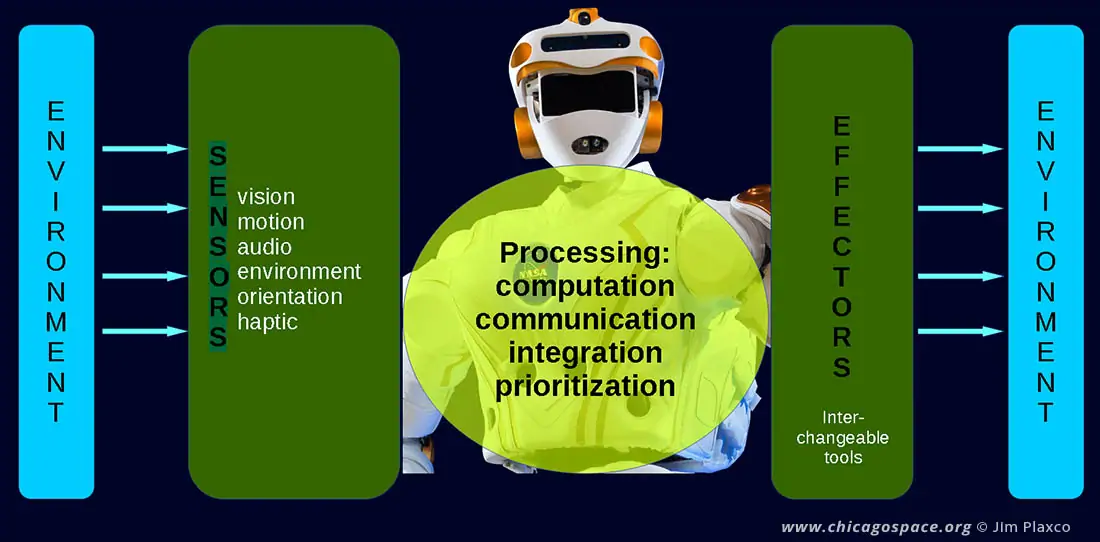
Machinery and robots are evolving and becoming ever more capable, versatile, and flexible whereas human evolution ended long ago. We humans are not going to sprout wings or grow rows of eyes on the backs of our heads – but our engineering prowess makes it possible for us to design machines with these characteristics if the need is there.
One aspect of the human vs robot debate that is relevant to this discussion is to look at robotic cost effectiveness with respect to today’s mining industry. In discussing the question of the cost of human missions versus robotic missions, we can begin by looking at what is happening here on Earth.
In the field of mining, the ARIDuA (autonomous robots and the Internet of Things (IoT)) in underground mining) project is working to merge robotics with interconnected networks of devices, appliances, and systems that can communicate and exchange data to provide enhanced capabilities. In an interview with NBC News, Dr. Bernhard Jung, a professor at Freiberg University of Mining and Technology in Germany, said that we may see “fully automated ‘man-less’ mines that are completely operated by machines,” and that with respect to mining in alien environments like the ocean floor or space “Making use of robots may be our only chance to ever extract minerals in such areas.” This observation correlates with an observation about asteroid mining made by Professor Scott Dunbar, head of the Norman B. Keevil Institute of Mining Engineering: “Asteroid mining is an interesting idea, since pursuing it leads to possible innovation in Earth-based mining. For example, we likely won’t send people to asteroids, so there would be a high reliance on robotics and autonomy.”(6)
The use of robotics and automated systems continues to replace human labor in manufacturing and mining with the expectation that the pace will continue. In a 2019 presentation about Made In Space’s Archinaut: a Construction Robot for Space, Jim Reuter, Associate Administrator of NASA’s Space Technology Mission Directorate, observed that “In-space robotic manufacturing and assembly are unquestionable game-changers and fundamental capabilities for future space exploration.” The lesson here is that improvements in robotic capabilities in one field, mining for example, can be applied to other fields, manufacturing for example.
Advances in artificial intelligence has defined deep deployment in manufacturing, and it can simplify the design and iteration process. In a presentation on the subject of artificial intelligence and manufacturing at the April 2023 Manufacturing For Growth Meeting, Jeff Ahrstrom, Ingersoll Machine Tools CEO observed that:
Imagine when, not if, a system develops and redevelops a process based on variables such as materials, tooling, machinery used, condition of equipment and environment, work holding available and design intent. Imagine the same system, iteratively, refining part characteristics and processes within the design intent.
Related to the above is the observation made by Werner J.A. Dahm, United States Air Force Chief Scientist (AF/ST) in the report Report on Technology Horizons A Vision for Air Force Science and Technology During 2010-2030, May 2010:
Although humans today remain more capable than machines for many tasks, by 2030 machine capabilities will have increased to the point that humans will have become the weakest component in a wide array of systems and processes. Humans and machines will need to become far more closely coupled, through improved human-machine interfaces and by direct augmentation of human performance.
Conclusion: The continuous evolution of networking, AI algorithms, sensor technology, haptic systems, and computing power combine to increase the capabilities of robotic and autonomous systems with respect to human performance on a wide range of tasks that require physical labor and decision making capability.
Conclusion
Space, be it orbital space or the surface of the Moon or Mars, is not life’s natural environment. There are substantial challenges to supporting a space-based human workforce. In today’s economic environment, automation and robotics and AI are becoming ever more cost effective and are replacing human labor in an ever widening variety of tasks.
Robotic advantages are far more pronounced when the environment is space. It is for this reason that projects like space solar power, lunar mining, asteroid mining, and zero-g manufacturing have the greatest chance of success if the development of the necessary space infrastructure focuses on the development and deployment of advanced robotic systems to carry out the necessary labor.
This is not to say that people have no business in space. Nor is it to say that we shouldn’t pursue space settlement. We should pursue the development of space settlement but the direction taken must align with the specific objectives the project is attempting to achieve. This means that one challenge that space settlement advocates must convincingly address is this: if mechanized labor is replacing human labor in humanity’s natural environment, and is known to be even more cost effective in the alien environments of space, then what crucial role does the establishment of space colonies serve?
References
(1) Pilgrim Fathers, Mormon Pioneers, and Space Colonists: An Economic Comparison (1) by Freeman J. Dyson, published in Proceedings of the American Philosophical Society, Vol. 122, No. 2 (Apr. 24, 1978),pp. 63-68
(2) Humans to Mars Will Cost About “Half a Trillion Dollars” and Life Support Roughly Two Billion Dollars, Harry W. Jones, NASA Ames Research Center, 46th International Conference on Environmental Systems, 10-14 July 2016, Vienna, Austria
(3) The Fourth Industrial Revolution, Klaus Schwab, World Economic Forum, 2016
(4) Automation, Labor Share, and Productivity: Plant-Level Evidence from U.S. Manufacturing, Emin Dinlersoz and Zoltan Wolf, U.S. Census Bureau, 2018
(5) Are Astronauts Worth Tens of Billions of Dollars in Extra Costs to Go to Mars?, https://theconversation.com/are-astronauts-worth-tens-of-billions-of-dollars-in-extra-costs-to-go-to-mars-111348
(6) Space: the Final Frontier of Mining – Exploring Space-Based Resources, Connect, Volume 23, 2018, Teck, https://www.teck.com/news/connect/issue/volume-23,-2018/table-of-contents/space–the-final-frontier-of-mining-
Jim Plaxco is President of the Chicago Society for Space Studies and serves as the National Space Society’s Space Ambassador Program Subject Matter Expert for Economics.