by Mike Combs, Copyright © 2022
One of the most outstanding advantages orbital habitats have over planetary colonies is while the latter would be settlements in one particular location, space habitats can be built anywhere in the solar system we want to be. One of the few limitations is the orbit must not be frequently eclipsed by a planet. Even that requirement may apply more to space habitats as Gerard O’Neill originally envisioned them, with giant mirrors reflecting natural sunlight into the interior, than to the LED-illuminated kind people are discussing more lately.
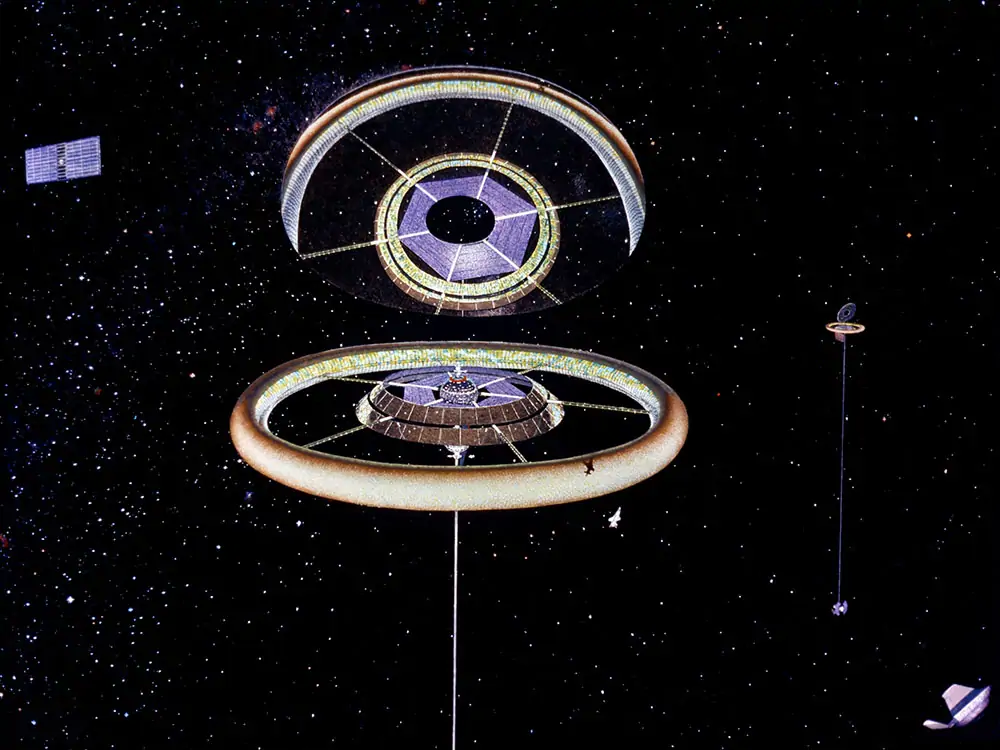
NASA art of the exterior view of a Stanford Torus space colony hosting a population of 10,000. Image Source: NASA, Art work by Don Davis
While O’Neill habitats can orbit almost anywhere, it’s still the case that some orbits would be more advantageous than others. Where is the prime orbital real estate in our solar system?
One of the primary factors in this series of comparisons will be local availability of resources. We’ll also consider the geometry of space-time for advantages. In this analysis, we’ll start close in to the sun, and range further and further out.
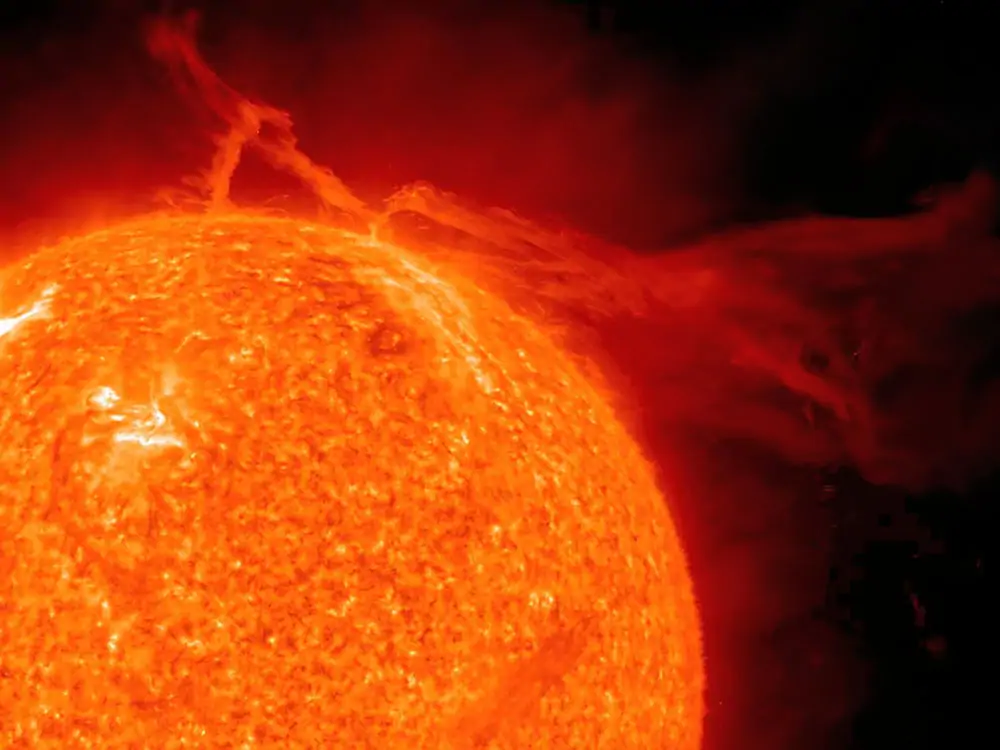
Sol, our Sun and the eruption of a solar prominence as seen in ultraviolet light. Image Source: NASA
Orbits inside that of Mercury’s
There’s not much advantage here. It’s hypothesized there might be a class of asteroids called Vulcanoids, which could be a source of construction materials. Thus far they remain theoretical (observations this close to the sun are difficult).
An advocate might say an advantage for any orbit closer to the sun than Earth’s is that solar levels would be higher. The specific advantage implied is the mirrors and photo-voltaic arrays of an orbital habitat could be somewhat smaller. That’s not a compelling economic argument for the following two reasons. The mirrors and PV arrays would be of very simple, repetitive construction. This means, with a bit of automation, construction costs should be low. Also, these zero-gravity structures would be of very flimsy construction, holding down material costs.
Celestial bodies orbiting inside Earth’s orbit are very desiccated, with limited supplies of the volatiles space habitats will need. Between the issues of required mirror size and availability of volatiles, the latter will be the trumping economic factor.
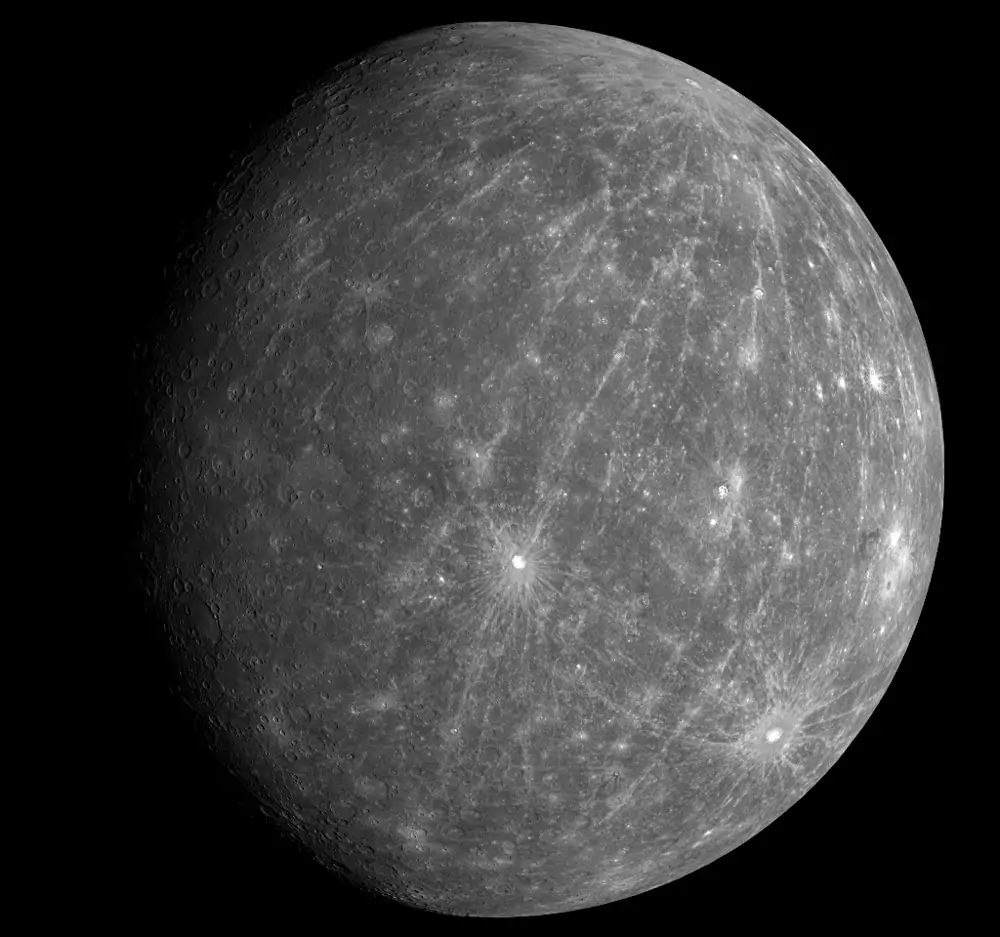
The innermost planet Mercury featuring Kuiper Crater as revealed by the Messenger (MErcury Surface, Space ENvironment, GEochemistry, and Ranging) spacecraft. Image Source: NASA
Mercury
If Mercury were sun-locked (as astronomers originally thought), we could talk about a mass-driver at the center of the sunward side (where solar power would be at its most intense) firing ore to the Sun-Mercury L-2 point. However, it’s been known for some decades now that the rotation of Mercury is not sun-synchronous. This means no location on the surface of Mercury gets continuous sunlight from overhead, and unlike the case with our moon there’s no location where a single mass-driver can launch continuously to a convenient L-2 point. Perhaps one could have a series of mass-drivers spaced around the equator of Mercury being used sequentially, but that would multiply expenses.
Therefore, space habitats orbiting Mercury, while not impossible, may remain unlikely.
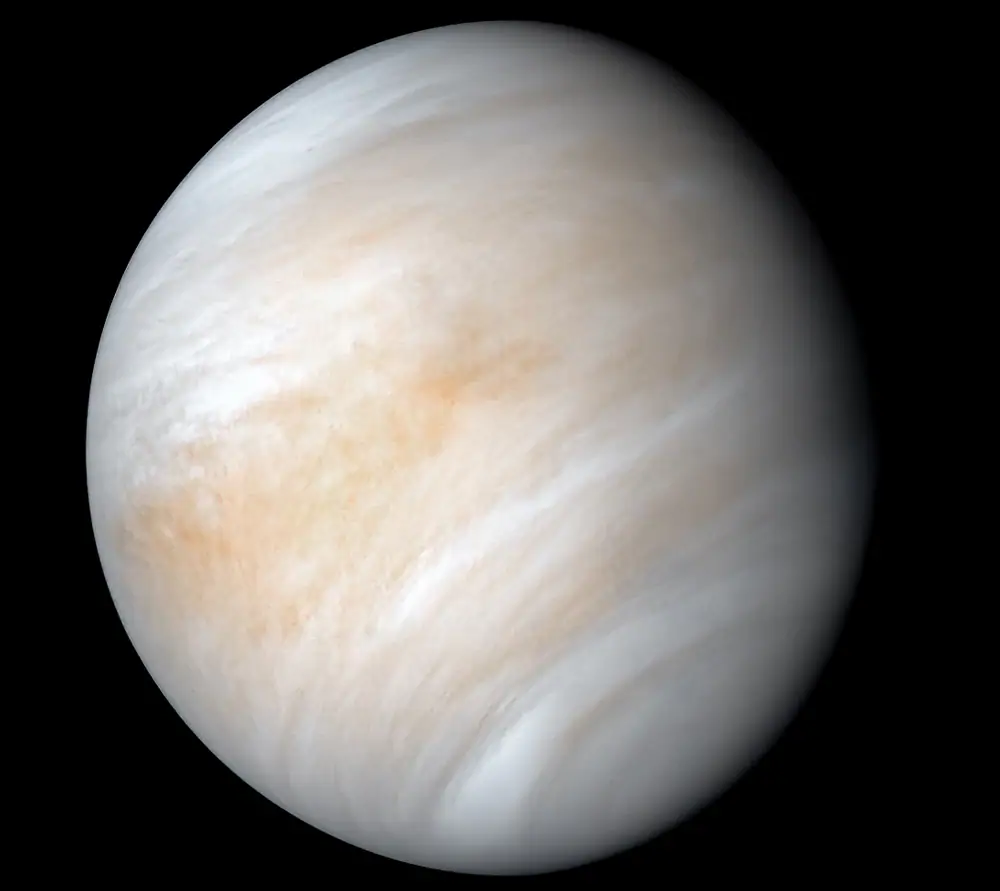
The planet Venus as photographed by NASA’s Mariner 10 spacecraft. Image Source: NASA
Venus
Both prospects and motivations seem poor here.
Venus is moonless, and due to extreme conditions on the surface could not itself be counted on as a source for raw materials. Conceivably, scoop ships might harvest CO2, but orbital habitat construction will require more than just carbon and oxygen.
It’s hard to see motivations for wanting to live in orbit of Venus. The view is not as attractive as that of Jupiter or Saturn. Venus can be scientifically examined from orbit, but that doesn’t require humans onsite. It’s doubtful there’ll be any human surface explorations in the foreseeable future.
It’s been pointed out by some that breathable air is a lifting gas in the atmosphere of Venus. Such balloon colonies might be built someday just for the sheer novelty. I think even if such settlements are technically possible, they would be economically uncompetitive due to the resource-availability issue and difficulties with export.
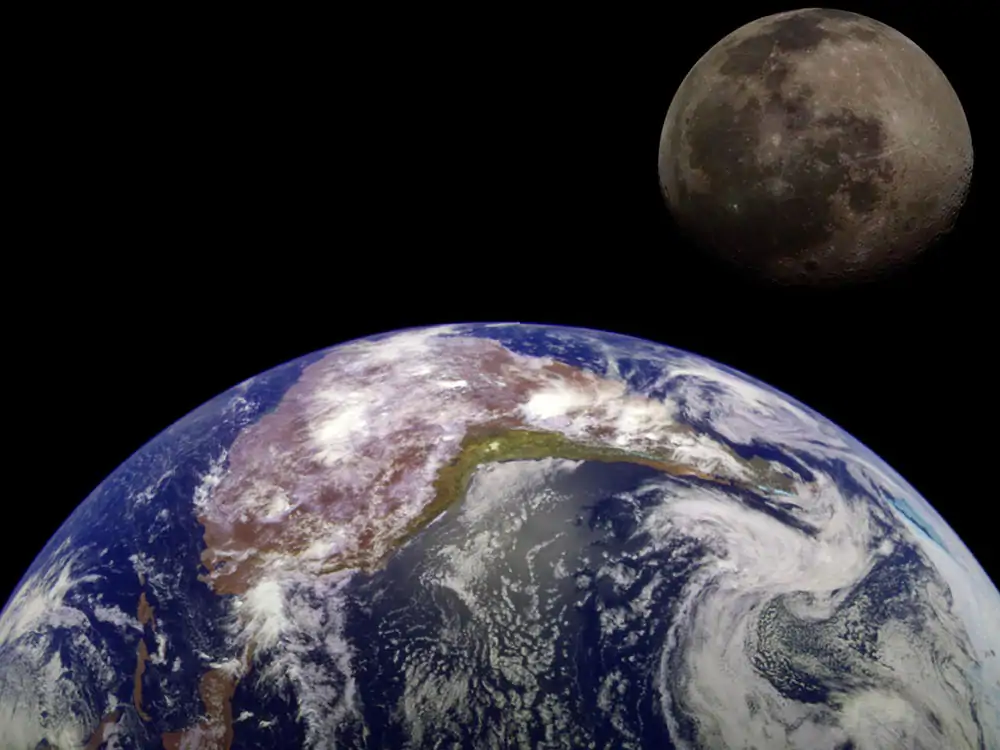
In 1992, the Galileo spacecraft returned images of the Earth and Moon. The two images of the Earth and Moon were combined to generate this view. Image Source: NASA
Earth orbits
This is certainly where space settlement will begin. It’s just barely possible the first O’Neill-class orbital habitat will be built by sending mining equipment, ore refineries, and fabrication/construction facilities to a Near Earth Asteroid, with construction taking place in orbit around that asteroid. However, there’s an important issue to consider beyond just the delta-V increment between the source of materials and the construction site.
At the beginning of the process, all available markets will be in the spaces immediately surrounding Earth. Economics points to the enterprise starting here. It’s been argued this is a reason to expect orbital settlement to begin before large-scale Mars settlement.
The Earth-moon L-5 point still gets lots of press. Many space settlement advocates seem not to know (or have forgotten) that L-5 was the first but not final word on where our first space manufacturing facilities and habitats should go.
The issue is that the orbital location chosen must split the difference between having a modest velocity difference to the source of building material and having easy access from Earth.
The High Frontier studies initially pointed to L-5. But after what O’Neill called “a brief flirtation” with a 2:1 resonant orbit, the studies eventually settled on a circular high orbit about half the distance of the moon’s orbit (typically referred to as High Earth Orbit).
There’s a lot of room for habitats in this orbit. In my opinion, it would get crowded politically sooner than literally. At that point, kidney bean-shaped orbits around not only L-5 but L-4 as well would be additional options (the choice of L-5 over L-4 was rather arbitrary).
One might ask about orbits around the moon. Close orbits are not advised. Gateway is not the first proposal for a space station closer to the moon. The first dates back to Apollo, and was called LOSS: Lunar Orbiting Space Station. Turns out the problem with low lunar orbits is the moon is lumpy, resulting in irregularities in its gravitational field. These would cause slight but steadily-accumulating perturbances to the orbit, ending with the low point of an orbit coming below a high point of the lunar surface. This realization prompted one of the researchers involved to remark, “LOSS would have been a true loss”.
Instead, Gateway will go into a near-rectilinear halo orbit. There’s a disadvantage to halo orbits around L-2, L-1, or L-3. They’re only stable on 2 out of 3 axes, while L-4 and L-5 are stable on all 3.
Before leaving the subject of orbits around Earth, we should look at the work of Joe Strout and Al Globus. Most of this article is concerned with space settlements as originally conceived by Gerard O’Neill. One of his assumptions was enormous space mirrors reflecting sunlight in through large windows. Thus, it becomes a design requirement that a habitat orbit cannot be frequently eclipsed by a planet.
More recent thinking suggests that artificial illumination of the interior with LEDs might be sufficient. Quality of life issues will eventually lead us back to the natural sunlight designs of earlier proposals. However, it must be granted that artificial lighting frees us from the constraint that habitat orbits must avoid frequent eclipses. Lower Earth orbits with lower delta-V increments with the surface become possibilities.
Strout and Globus have pointed out that Equatorial Low Earth Orbit (ELEO) enjoys much better geomagnetic protection from space radiation than low orbits with higher inclinations (like that of the ISS). Add to this an equatorial spaceport on Earth, and you have a winning combination. Since 9/10th the mass of a space habitat might be the radiation shield (and higher spin rates reduce the required diameter), they feel such ELEO habitats might even precede the era of space mining. They’re certainly describing the genesis of the orbital habitat progression.
Turning now from nearer to the Earth to further away, we could consider habitats lying directly on Earth’s solar orbit moving in lockstep with it. The amount of room for habitats in this orbit is staggering. When this orbit is well-populated, joining each habitat to its neighbor could form a linked arc, enabling easy travel throughout. Placing the ends of this arc inside the Sun-Earth Lagrange points L-4 and L-5 might promote overall stability.
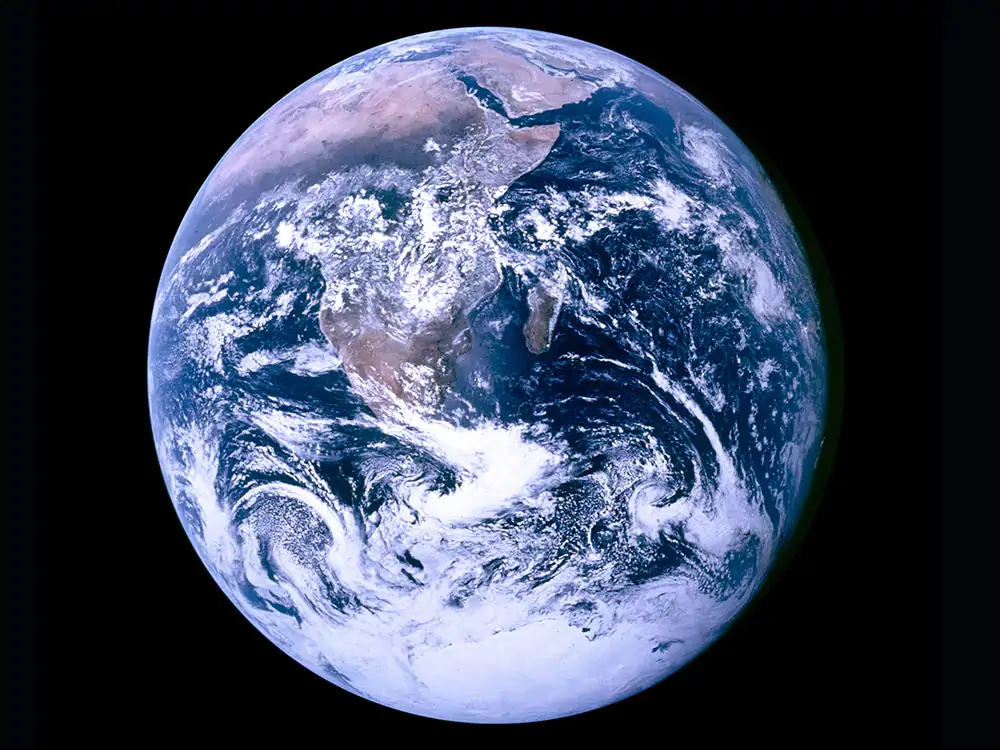
The Blue Marble – Our Home Planet – Earth. Image Source: NASA
Orbits adjacent to Earth’s orbit
Solar orbits which are close to but independent of Earth’s orbit might be possibilities. We would want to minimize gravitational disturbance from a passing planet, so we could consider an orbit about halfway between the orbits of Venus and Earth. Outside Earth, the orbit with the least disturbance could be significantly beyond the half-way point to Mars orbit, since Mars is a smaller planet with less gravity. It would be a much bigger orbit than the previous. The economic principle that greater availability of volatiles outweighs greater insolation suggests the latter orbit is the preferable one. Near Earth Asteroids would be the source of building materials.
It should be noted that while either of these orbits would have a modest delta-V to Earth, the launch windows would be very infrequent.
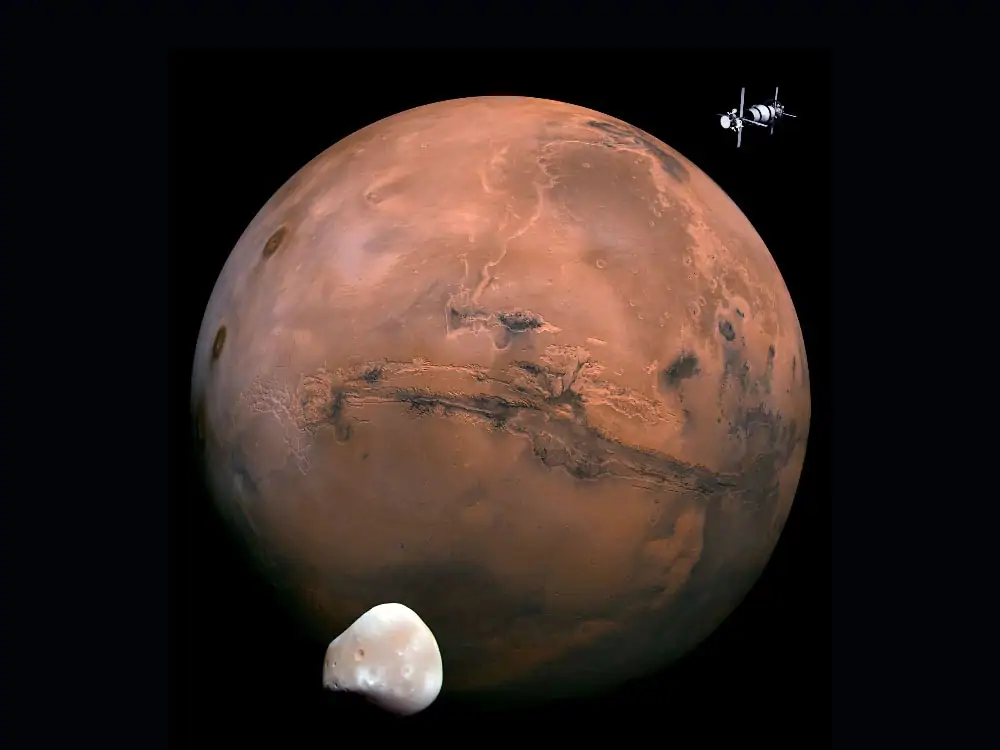
A composite image of Mars (the Red Planet), the Martian moon Deimos, and a Bernal Sphere space colony. Image Sources: NASA
Mars
In my short story Parable of the Red World, I mention a space habitat being built in orbit of Mars from raw materials harvested from the upper moon Deimos. Since Deimos is just above Aresynchronous orbit, that seemed a sensible orbit for the settlement. Here, a habitat would be eclipsed by the planet a bit more than in the previously-mentioned ideal High Earth Orbit. But since this is a synchronous orbit, and since anyone living in the Mars system would doubtless be operating on Martian time, eclipses could be scheduled to only happen at night.
Are there any reasons to construct an orbital habitat around Mars versus other solar system locations? The resources of Deimos would almost certainly be adequate to the task, but are hardly unique from asteroidal materials available from targets with much lower delta-V’s from Earth.
A Mars advocate might assert that certain raw materials are available on Mars in a form more conducive to extraction. But in space, the primary economic factor for resources will always be the export levy imposed by gravity. Lifting raw building material from gravitational fields of planetary magnitudes is to be avoided.
One reason for building habitats in orbit of the red planet might be you’re committed to a program of Mars surface exploration, but have found 1/3 G is insufficient to avoid long-term health problems. The delta-V increment between the orbital habitat proposed and the surface of Mars would be less than 6 km/sec (and you could refuel at both ends), so there could be a good deal of travel back and forth. Surprisingly, the biggest part of the surface exploration might take place from the habitat via telepresence. In a surface-synchronous orbit, you could even avoid the signal latency involved in routing through a Martian communication satellite.
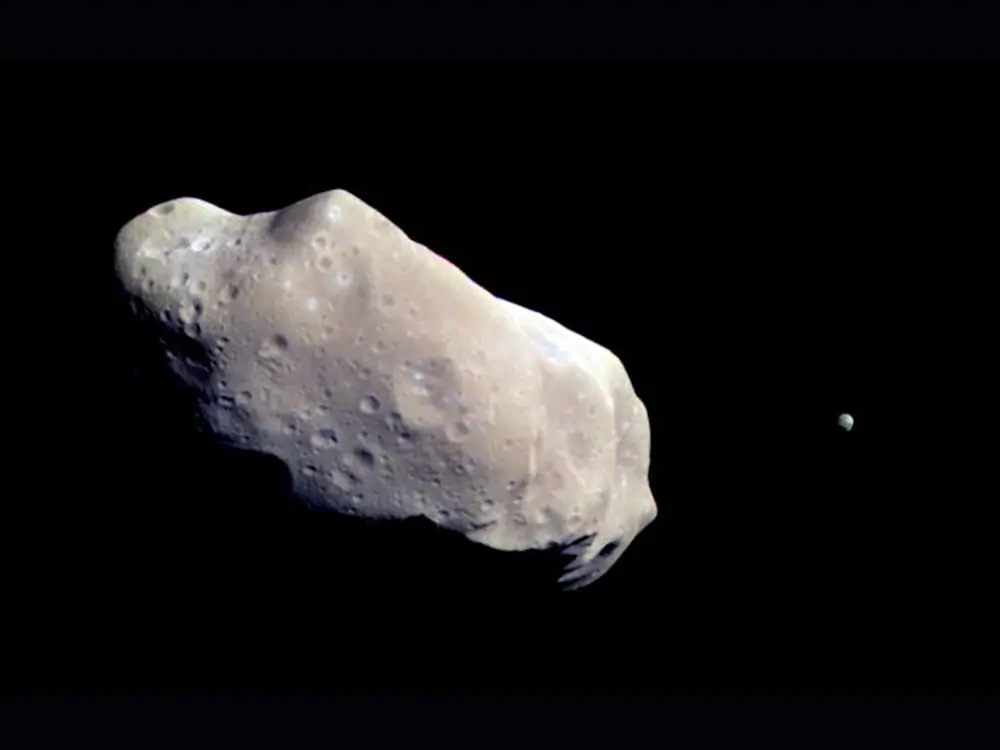
Asteroid Ida and its moon Dactyl as imaged by the Galileo spacecraft in 1993. Image Source: NASA
The Asteroid Belt
When we reach the point that Earth markets are no longer the dominating aspect of space habitat economics, the Belt is hands-down the most advantageous location for construction of orbital settlements. It contains all of the elements we need in small, bite-sized, easily-digestible nuggets.
One might ask why asteroids get so much attention when it comes to extraterrestrial mining. The reality is that most of the raw materials in a planet are unobtainable to us. The deeper you dig, the greater the pressure. (On planets with molten cores, like Earth, there’s also a buildup of temperature.) Asteroids have negligible gravity. Not only is it easier to get raw materials away from an asteroid, the increase in pressure as you tunnel deeper is negligible as well. Unlike the situation with planets or large moons, the entire mass is accessible for building material.
No doubt space settlements will be built in every conceivable orbit within and near the Asteroid Belt. One might question the “within” part. Wouldn’t it be better to orbit just inside or just outside the Belt to minimize the risk of catastrophic collision? In truth, the risk of meteoroid impact in space is greatly exaggerated in the public imagination (no doubt due to science-fiction TV).
Assuming we do want to talk about orbits just inside and just outside the Asteroid Belt, which would be most advantageous? Orbits in the inner part of the Belt would have lower round-trip delta-V’s with Earth, but this would be a very incremental difference. The outer parts of the Belt will have a greater abundance of water, and this will most likely be the deciding factor.
In time, the human population in the Belt could exceed both that of the Earth and the habitats orbiting it.

Redefined from being an asteroid to a dwarf planet, Ceres is the only dwarf planet in the inner solar system and is the largest object in the asteroid belt between Mars and Jupiter. Image Source: NASA
Ceres
In popular science-fiction depictions of a Belt civilization, Ceres is usually described as its capital or urban center. This might be the right idea, but for the wrong reason.
It may stem from planetary chauvinism: Ceres is the biggest asteroid, therefore the most like a planet (it’s even round), so surely, it’s the most inhabitable. But in the High Frontier strategy even small asteroids can be the nucleus of a new orbital settlement operation.
Recent research notes large amounts of volatile materials available in Ceres (possibly more water in the form of subsurface ice than in all the oceans of Earth), and posits that this asteroid began life not in the Asteroid Belt but far beyond it, gradually moving to its current position. As such, it can be a valuable source of ammonia ice. This is important, as nitrogen is one of the elements of the ammonia compound, and nitrogen will be in high demand for habitat atmospheres.
Settlements built in orbit of Ceres may have enormous, open interior volumes since atmospheres can be less expensive. In some solar system locations, the torus might be a favored geometry for space habitats as it minimizes the volume of atmosphere required. Here, habitat designers might tend more toward spheres or cylinders. Space settlements constructed here might also feature large bodies of water, ranging from enormous lakes perhaps up to small seas.
So, Ceres might truly be prime Belt real estate, but not because it’s the biggest asteroid.
Pekka Janhunen has designed a Ceres-orbiting megastructure consisting of an enormous disk of interconnected cylindrical habitats. Janhunen’s work is top-notch, and I would only have one nit to pick. I would expect space habitats to get built adjacent to Near Earth Asteroids long before any asteroid in the Main Belt.
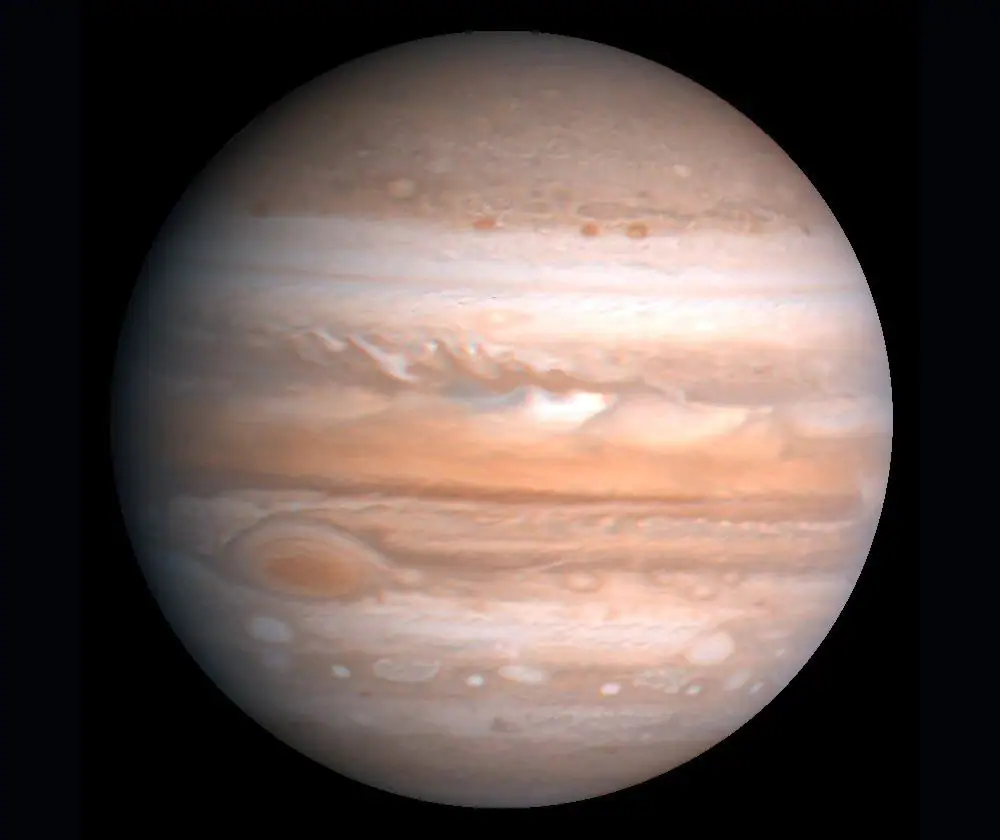
A color image of Jupiter created by the U.S. Geological Survey from a 1979 image taken by the Voyager spacecraft. Image Source: NASA
Jupiter
In discussions of the Jupiter system, the Galilean satellites get lots of attention. Might we want to build settlements in orbit of Io, Ganymede, Europa, or Callisto? It might be another example of planetary chauvinism to assume these biggest moons would be the best locations for operations. Of the four, only Callisto orbits largely outside of the deadly Jovian radiation belts.
In any case, the outermost smaller moons of Jupiter would be better sources of raw materials for space habitat construction. The outermost moons would be preferable because we should choose orbits outside the steepest parts of any gravity well, and that of Jupiter is very steep indeed.
While well-known for having some large moons, Jupiter also has dozens of small moons. The latter will prove to be of more interest to orbital homesteaders.
Ammonia ice will be more plentiful this far from the sun.
At the Sun-Jupiter L-4 and L-5 points are two arcing belts of asteroids called the Jupiter Trojans. These Trojan asteroids should be even better-stocked with volatiles than the Main Belt asteroids.
Is there another advantage to residing in the Jovian system? Jupiter has a deep gravity well second only to that of the Sun. High Frontier philosophy is to avoid deep pits in space-time. Although it might be disadvantageous to live near the bottom of a steep gravity well, one might still be useful.
In space travel there’s a principle called the Oberth Effect. Whipping around inside a steep gravity well while burning large amounts of fuel in the deepest part (where velocity is highest) can result in an extra boost of delta-V.
If this sounds suspiciously like getting something for nothing, consider what happens to the exhaust atoms. The fuel starts out in a position of high potential energy. Then when you blast those atoms out the engine they wind up in a lower, elliptical orbit with lower potential energy. This energy difference goes into greater kinetic energy in your vehicle.
Thus, there would be an advantage in fueling your spacecraft at a Trojan asteroid or one of the outermost Jovian moons, and then doing a close pass by Jupiter. Blowing out most of your fuel deep in that enormous gravity well you’d enjoy a Oberth Effect boost in speed. Returning spacecraft could do the maneuver in reverse to help slow down.
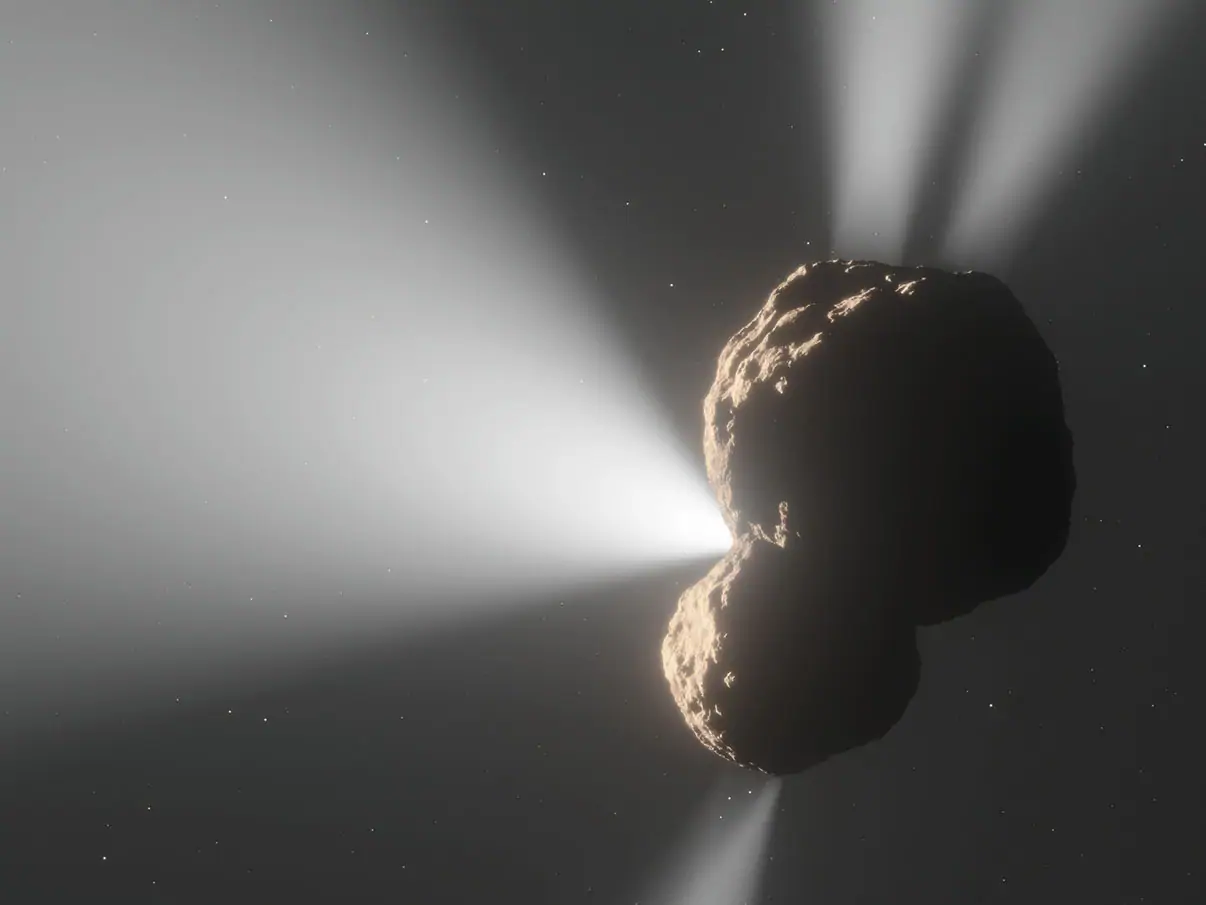
An artist’s concept of Centaur 29P/Schwassmann-Wachmann 1’s outgassing activity as seen from the side.
Image Credit: NASA, ESA, CSA, L. Hustak (STScI)
Centaurs
The Centaurs are defined as small asteroidal or comet-like bodies orbiting beyond the orbit of Jupiter but inside the orbit of Neptune. However, not all of them are tiny. Both Chariklo and Chiron are estimated to be well over 200 km in radius (and seem to be ringed). It’s also a vast population, with at least 44,000 bodies larger than 1 km in diameter.
Astronomers tell us the orbits of the Centaurs are unstable since they roam among the giants of the solar system. Of course, they mean unstable over astronomical timescales. It’s doubtful the builders of a space habitat will be worrying millions of years into the future.
Observations indicate the Centaurs are diverse in their compositions, suggesting a variety of materials would be available for mining.
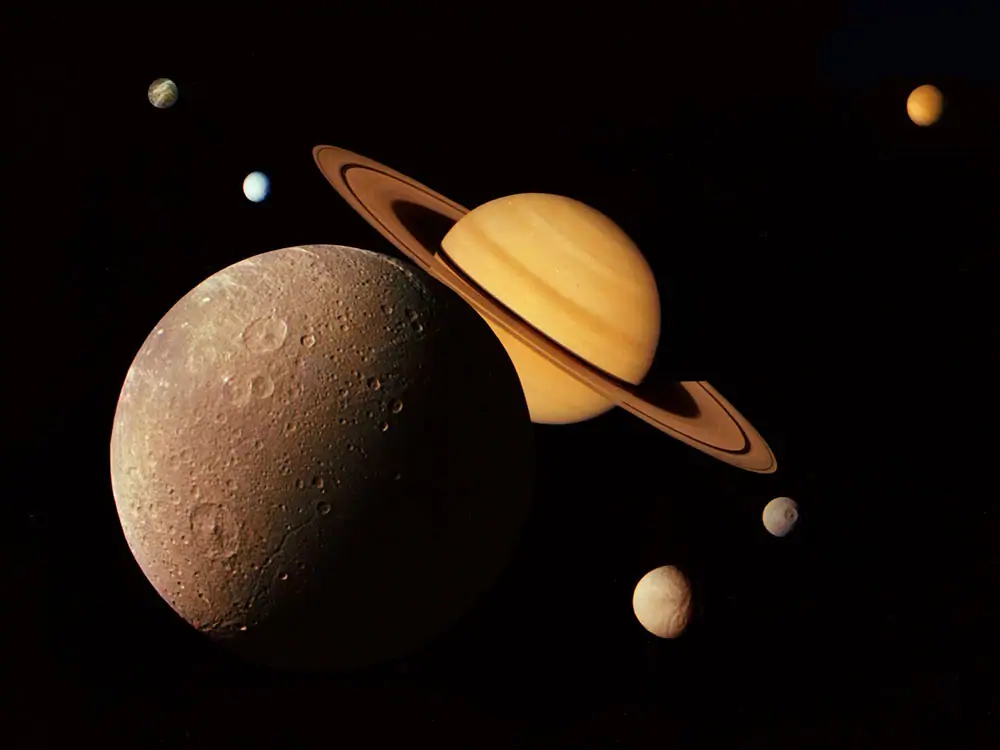
A photo montage showing Saturn, and its moons Dione, Tethys, Mimas, Enceladus, Rhea, and Titan. Image Source: NASA
Saturn
Saturn has less-intense radiation belts than Jupiter and the greatest number of moons of any planet in the solar system. Here, too, tapping into the outermost moons over the innermost ones is recommended in keeping with the principle of avoiding the steepest parts of gravity wells.
Enceladus (a moon of great interest to exobiologists) has water geysers spraying into space. Water might thus be obtained in liquid form by pipe wells of modest depth. This could be more advantageous than mining water in the form of ice from the surface. At these temperatures water ice differs greatly in its physical characteristics from that we know on Earth, having the toughness of granite.
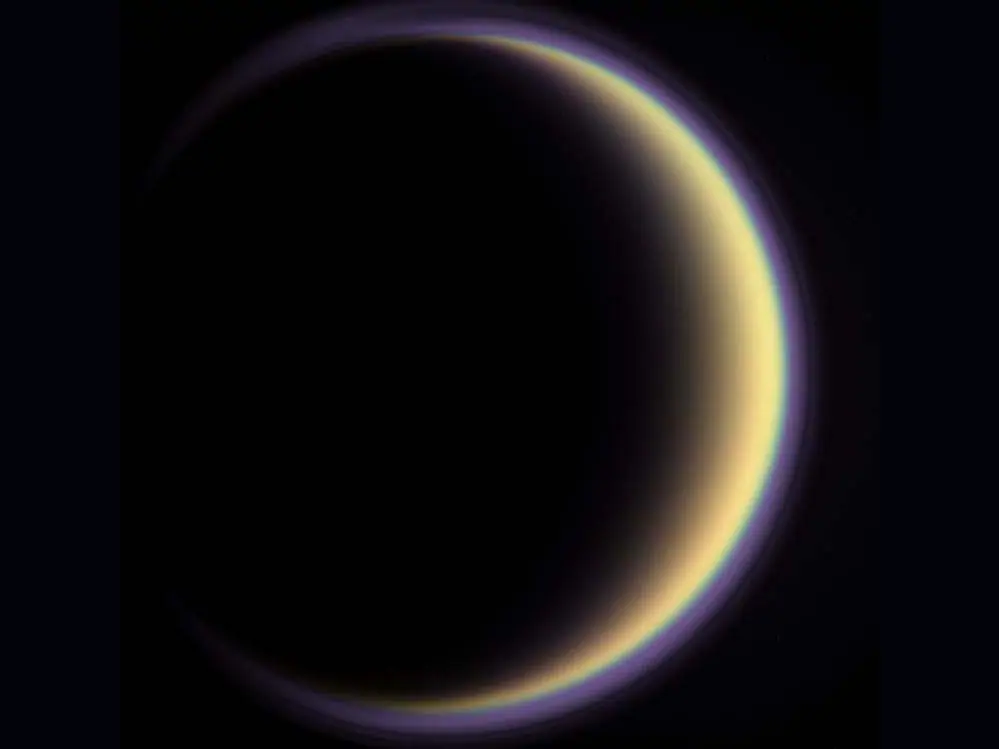
An image of Saturn’s moon Titan taken by the Cassini spacecraft. Image Source: NASA
Titan
Titan has an aspect which is very nearly unique in the solar system. It’s the only body other than Earth with a thick atmosphere composed largely of nitrogen. We’ve already established nitrogen will be an element in demand by space habitats. Might it be economically viable to retrieve it from Titan?
Titan’s escape velocity is only a bit more than that of Earth’s moon. However, the thick atmosphere might rule out mass-driver launch from the surface. Balloon-borne mass-drivers residing permanently in the upper atmosphere could be a viable alternative. In the oxygen-free atmosphere of Titan we could use hydrogen balloons free of concern for the explosive fire hazard they pose on Earth.
We might use jet engine vehicles on Titan with tanks of liquid oxygen inside the aircraft being burned with methane pulled in from the atmosphere. This would be an amusing reversal of the situation on Earth, with oxidizer being carried in the vehicle and fuel being pulled from the air. Nuclear-powered ramjets might be another possibility for Titan.
However, the best option for exporting nitrogen from Titan might be a space elevator. Spools of cable would be transported to the Saturn-Titan L-2 point, and cable would be played out in opposite directions toward and away from Titan. There might already be a space elevator emplaced on Earth’s moon by this point. The engineering would be much the same. One difference would be a thick atmosphere at the moonward end, but this should present no problem with the anchoring operation. Wind speeds in the cold atmosphere of Titan decline markedly as you approach the surface where the air has the most density.
The primary payload for this space elevator would be tanks of liquified nitrogen. Liquifying the nitrogen would be much easier in the frigid environment of Titan than on Earth. One could place the warm end of a heat-pump loop beneath one of the numerous methane/ethane lakes which dot the surface. Even modest temperatures on the heat radiator would make the liquid hydrocarbons of the lake boil furiously, providing a prodigious heat sink. Don’t worry about boiling away such a lake entirely. Titan has a hydrological cycle of evaporation, condensation, rainfall, and river flow into its vast hydrocarbon lakes.
So, the Saturn system might not be the worst place to build large-volume space habitats since atmospheres can be cheaper thanks to the low local cost of nitrogen. Another motivation for wanting to live in the Saturnian system might well be the view. If built adjacent to a moon not overly distant, a habitat could hardly have a nicer view than that of Saturn and its complex ring system.
One might talk about harvesting ice from that ring system, but there are two strong arguments against that, one practical and the other aesthetic. Better to obtain needed resources from much higher in the gravity well. And who would want to even incrementally diminish one of the loveliest spectacles of the solar system?
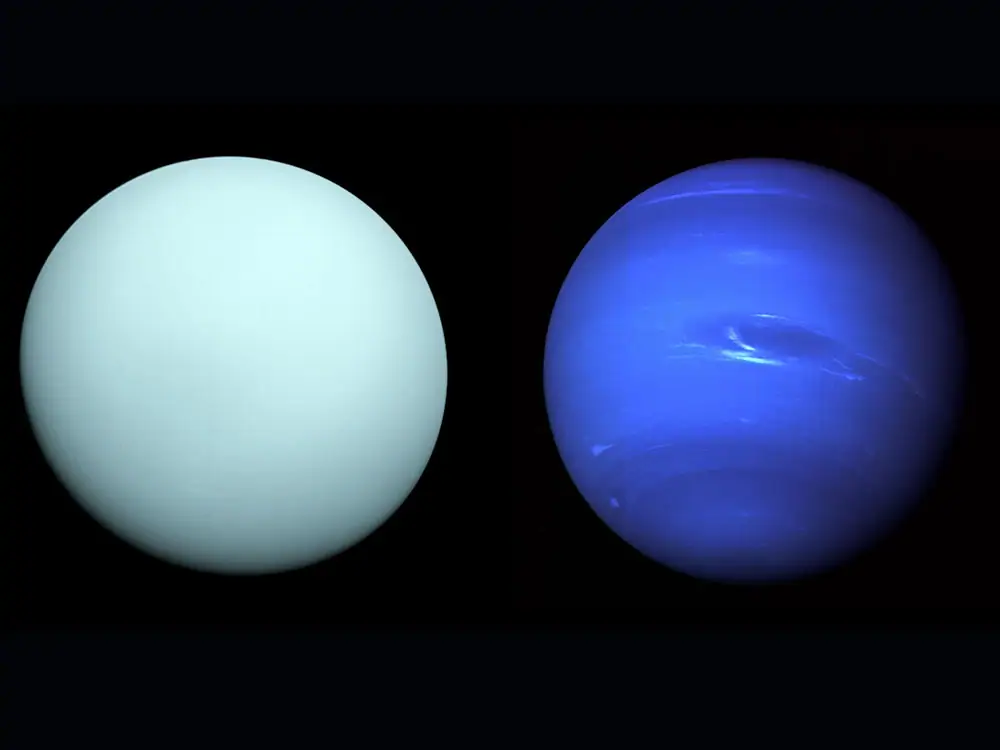
Uranus (left) and Neptune (right) as imaged by the Voyager 2 spacecraft. Image Source: NASA
Uranus and Neptune
As with the gas giants, these two ice giants have small moons which are rich in ice. As before, smaller moons farther out from the planet are most efficient to mine or live in orbit of.
We’re now sufficiently far from the heat of the sun that nitrogen can exist as ice. We would harvest this in preference to ammonia ice since it would save us the step of separating the nitrogen from the hydrogen in ammonia.
The Neptunian moon Triton has geysers of gaseous nitrogen, but it may not necessarily be more economical to harvest from this source via gas wells than simply mining frozen nitrogen from the surface. Nitrogen ice at these temperatures has a consistency close to that of toothpaste, and thus may actually be easier to mine than the granite-hard water ice.
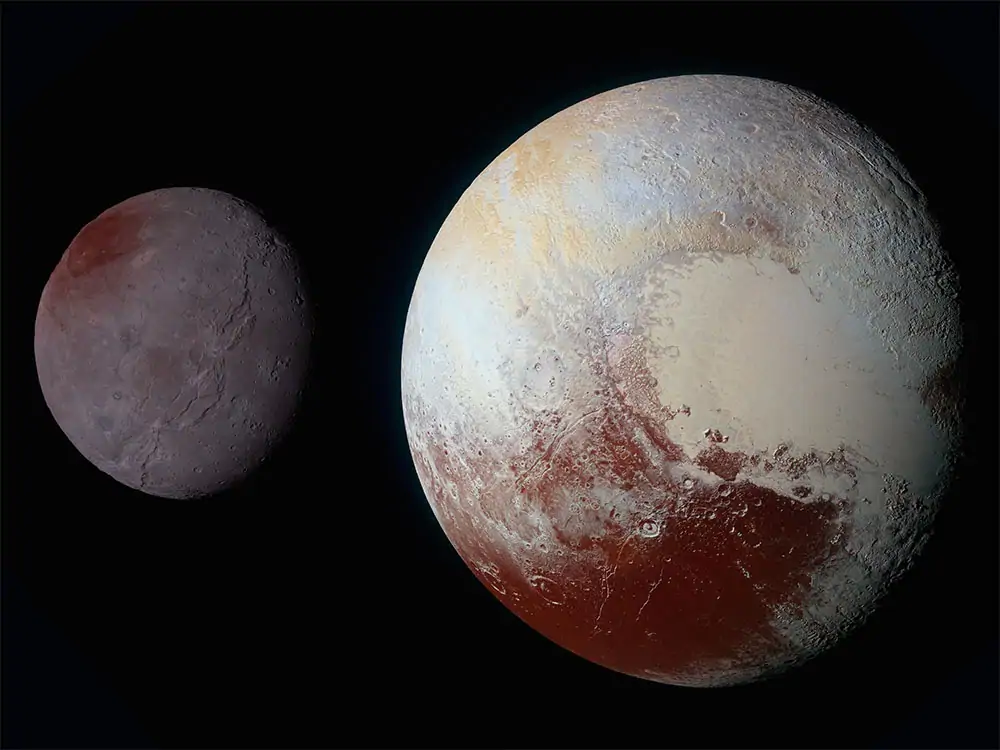
Dwarf planet Pluto (right) and its moon Charon (left) in a processed image taken by NASA’s New Horizons spacecraft in 2015. Image Source: NASA
Pluto and the Kuiper Belt
The Kuiper Belt does not get as much attention as the Main Asteroid Belt. However, it’s much richer in ices and nitrogen than is the main belt, though much more distant, extending from just outside the orbit of Neptune to beyond Pluto. In fact, Pluto is merely the most famous resident of the Kuiper Belt, and shares it with a number of other, somewhat smaller dwarf planets.
Pluto and its moon Charon orbit each other with the barycenter of the system above the surface of Pluto. Each is tidally-locked to the other. Thus, it should be possible to build a cable transit system between the two, and such a system could be constructed with existing building materials. This would not only ease travel between the bodies, it would also simplify lifting raw material from either body. However, this may be solving a minor problem. The escape velocity from Pluto is a mere 1.21 km/sec, and Charon an even more modest 0.59 km/sec. There are also smaller moons in the Plutonian system with even less gravity.
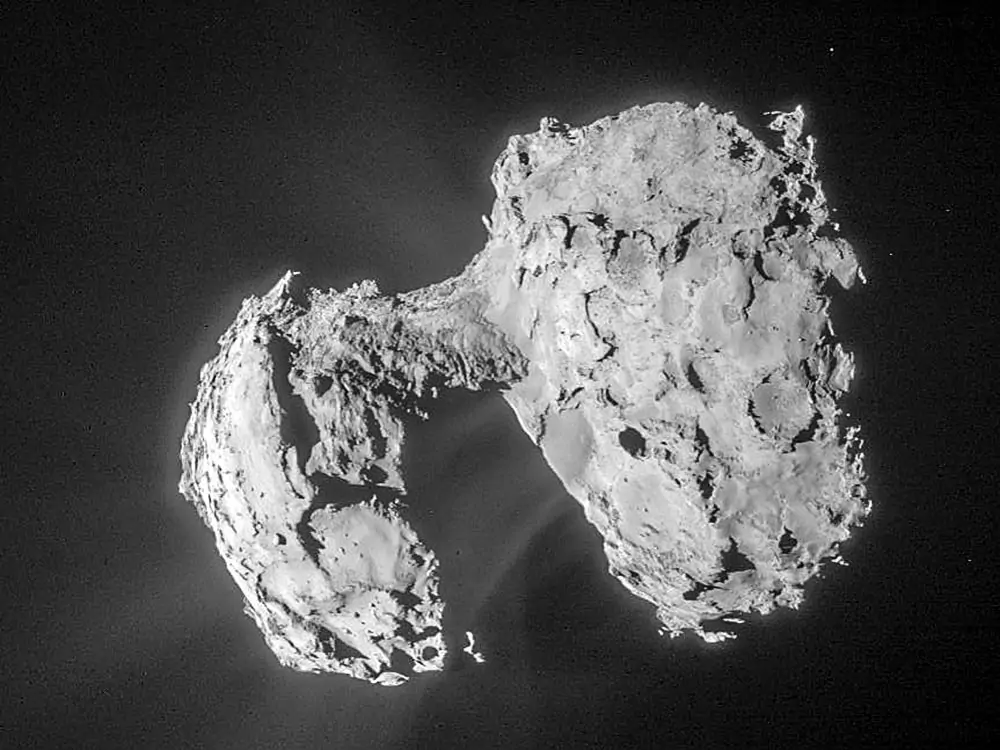
Comet 67P/Churyumov-Gerasimenko as photographed in 2015 by European Space Agency’s Rosetta spacecraft. Image Source: ESA
The Oort Cloud
Extending from about 2,000 to 200,000 AUs lies the Oort cloud of cometary bodies.
The comets we mostly discuss, called short-period comets, come to us from this cloud, having been disturbed from their circular orbits into much more elliptical ones ranging into our part of the solar system.
Someone may choose to build a space habitat adjacent to a short-period comet, but such a habitat would be economically disadvantaged. The highly-elliptical orbit would make travel to other solar system bodies expensive. The changing amounts of sunlight received would necessitate variable-geometry mirrors. These mirrors would enlarge their collecting surface area when further from the sun and contract it when closer.
Such solar mirrors would certainly be possible (perhaps for traveling habitats filled with citizens inclined to take in all the sights of both the inner and outer solar system), but would be more expensive to build and maintain. For my money, just stick to circular solar orbits (in this case remaining always within the Oort Cloud) and fixed-geometry mirrors.
The late Princeton physicist Freeman Dyson wrote quite a bit about living in the Oort Cloud, even dreaming of genetically-engineered “comet trees”.
A usual part of speculations about human communities in the Oort Cloud is they would be fusion-powered, as the sun would appear as merely an unusually-bright star. Surprisingly, solar power is still an option even at this distance. In zero gravity and vacuum, concentrating mirrors the size of states or even continents are possible and would mass much less than you’d tend to think.
Thus, there’s no reason for humanity to shrink back from the darkness of the outer reaches.
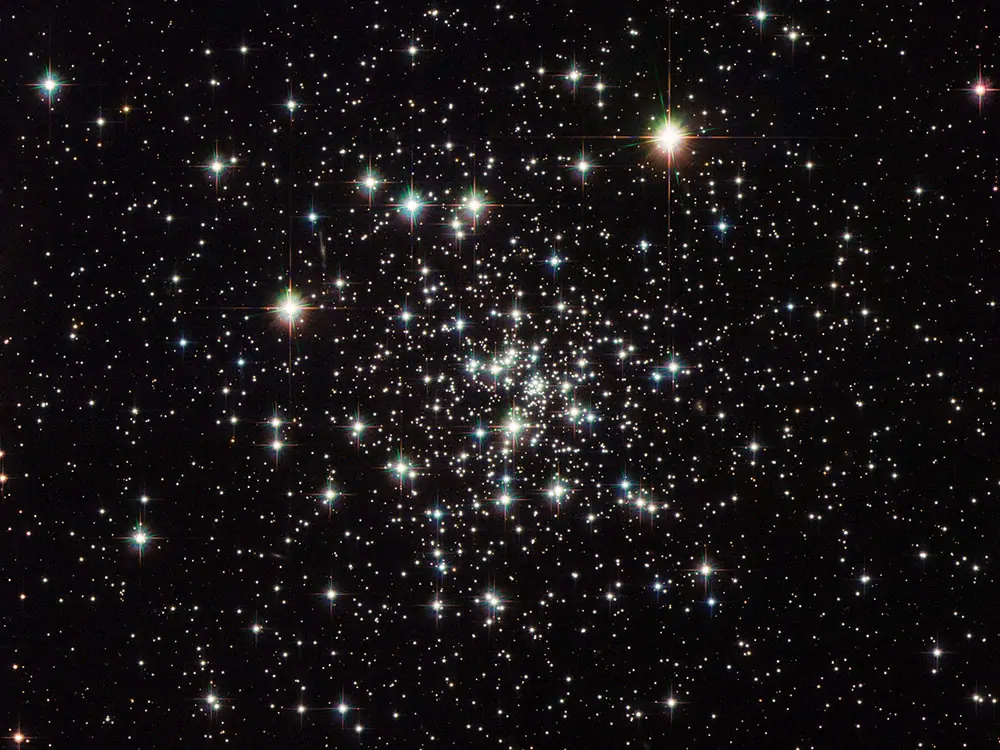
A Hubble Space Telescope image of NGC 6535, a globular cluster 22,000 light-years away Image Credit: ESA/Hubble, NASA
Interstellar
When it comes to interstellar travel technology, our order of preference would be:
- Jump drives
- FTL drives
- Relativistic drives
- Sleeper ships
- Generation ships
Even if the technologies of the first four remain forever out of reach, the space habitat concept enables comfortably-roomy generation ships. The only significant change is transitioning from solar power to taking your power supply with you.
Starships on this scale would be an answer to the biggest objection raised to the generation ship concept: What right do we have to condemn descendants to a lifetime aboard a ship? If a starship is on the scale of an aircraft carrier (and even with as pleasant interiors as the Galaxy-class Enterprise), this objection might have merit. But if someone born and living their entire life on a generation ship could fish in a stream, stroll through a forest, swim in a lake, climb a cliff wall, or hang-glide, they could hardly be viewed as deprived.
An interesting point has been made about orbital habitats built in the Oort Cloud. It’s pointed out that comets occasionally get disturbed in the other direction, getting flung out of our solar system. Additionally, there’s every reason to expect that other solar systems have Oort Clouds of their own. Those of very close stars might even overlap slightly with ours. With no deliberate decision on their part, some cometary residents might eventually become inter-system travelers. Such journeys might take millions of years, but that might not be an issue to people using fusion power anyway and with a few megatons of ice coming along for the ride.
This has been referred to as “interstellar osmosis”. Nevertheless, it’s doubtless most interstellar crossings will be more intentional, and will at most take centuries rather than many millennia.
A fascinating aspect of the orbital space habitat concept is that any solar system becomes a candidate for settlement. When we confine our thinking to planetary settlements, we find only certain solar systems with certain types of planets remain on the list of suitable targets. With High Frontier technology in hand, even red dwarfs (much more abundant than our type of sun) and blue-white supergiants (much more energetic) become candidates for colonization. Color filtration could adjust the sunlight in habitat interiors to what we humans and other Earth life are presently used to.
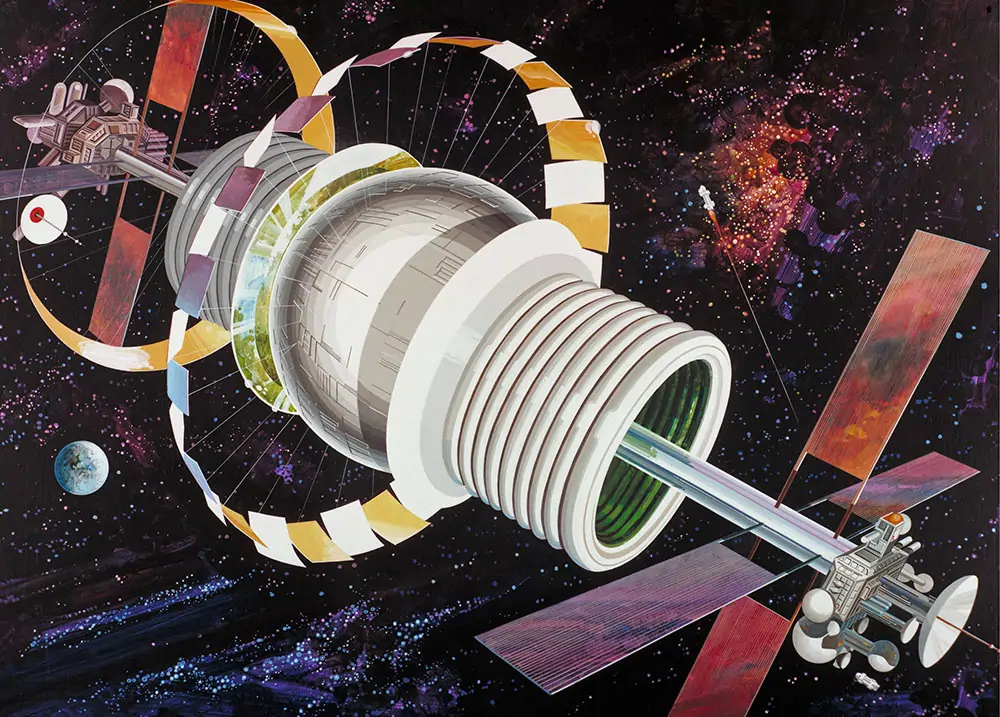
Artist concept of an exterior view of a Bernal Sphere space colony, home to a population of 10,000. Image Source: NASA
Summation
While various locations will vary in economic advantage, we can see there’s an abundance of orbits throughout our solar system and beyond for placement of settlements built from nearby space resources. This is an approach which expands our potential for living throughout the universe far beyond anything we more-typically envision.
Return to Mike Combs Space Settlement Collection Index
This content is a part of the Mike Combs Space Settlement collection and is provided as a courtesy of the Chicago Society for Space Studies and Mike Combs.